BEHAVIORAL NEUROPHYSIOLOGY LAB (Yangkyun Oh Lab)
Research Interest
Behavioral Neurophysiology Lab (Oh Lab) at Ewha Womans University

Deciphering the function of the metabolite sensors mediating the microbiome-gut-brain axis
Aim 1. To determine how the gut microbiota affects the activity of nutrient-sensing cells
Figure 1. A schematic for finding the gut microbial factor regulating the activity of nutrient-sensing neurons in the brain.
Controlling food intake, which is regulated by nutrient-sensing neurons and other feeding control neuro-circuits, is essential for preventing metabolic diseases. Those nutrient-sensing neurons are regulated by various upstream peripheral signals, which convey information regarding the internal energy status. Although the delicate control of the nutrient-sensing neurons is crucial for maintaining balanced food intake and energy homeostasis, understanding the upstream signals that regulate the activity of the nutrient-sensing neurons is still limited. Since the intestinal commensal microbial changes indicate dramatic internal metabolic changes, animals need to shift their feeding behavior and metabolic process to adapt to a sudden environmental change. Intriguingly, I found that the increased microbe species diversity in the gut reduced the amount of food intake in flies, indicating that the metabolites from the various gut microbes may regulate the neuronal circuit that controls feeding behavior. Combining these results, I hypothesize that the signaling cascades driven by the metabolites made by gut microbial species control the feeding behavior by modulating the activity of nutrient-sensing neurons. I further propose to test the idea that those metabolites can directly control the activity of the nutrient-sensing neurons and evoke the behavioral shift (Figure 1). To confirm this hypothesis, I plan to establish an axenic (germ-free) fly line in which intestinal microbes have been removed. Using this as a system, I propose to check the overall baseline activities and the glucose responses of the nutrient-sensing neurons in the brain, including CN, DH44, and a population of insulin-producing cells. Previous studies reported that the gut microbial community of Drosophila contains 5-20 bacterial species. Using an advanced bacterial engineering approach, I will introduce several species of gut microbes and test the activity of nutrient-sensing neurons to identify microbes that affect the activity of those neurons. Then, I will narrow down a specific enzyme that makes the candidate metabolites that control nutrient-sensing neurons’ activity. A successful outcome will reveal the neuronal mechanism by which the specific gut microbial factors influence nutrient-sensing neurons’ activity, thereby modulating food intake and metabolic balance.
Aim 2. To identify the intestinal metabolite-sensing cells detect the gut bacterial factors
Figure 2. A schematic of identifying a novel intestinal sensor cell that detects the metabolites made by gut microbes.
“All the diseases are from the gut,” Hippocrates said, to emphasize critical functions of the intestinal system in health and disease. The intestinal system is crucial for sensing and absorbing nutrients, vitamins, and essential metabolites and secreting various hormones that impact the overall metabolism in mammals and flies. Our previous study has shown that a population of the midgut responds to essential amino acid deficiency by secreting the CNMa neuropeptide. Upon release, CNMa signals to the brain to increase the protein-enriched food preference in flies (Kim et al., 2021, Nature). During this study, we also found that a commensal Acetobacter provides dietary leucine and isoleucine, which suppresses the secretion of neuropeptide from the gut even when flies are reared in a protein-deprived diet. Although this result suggests the existence of a collaborative metabolic network between gut microbiota and nutrient sensors, it is largely unknown how the gut microbes are involved in the regulation of intestinal nutrient or metabolite sensors. In addition, it is largely unknown which types of intestinal cells can work as sensors to detect distinct types of circulating chemicals from the gut microbes. To address these questions, I plan to examine my hypothesis that a specific population of the cell types of the fly midgut detects the metabolic changes and responds to adapt to the altered gut microbial changes. Then I will identify the species of the commensal microbes that collaborate with those midgut metabolite-sensing cells to optimize the response to internal nutrient perturbations, which evoke metabolic challenges (Figure 2). First, I plan to identify the specific intestinal cells that detect the metabolites made by gut microbes, including short-chain-fatty acids, polyamines, secondary products of amino acids, and bacterial vitamins. Then, I will characterize how those intestinal sensor cells transfer the metabolic information to the brain, fat body, and other organs. Additionally, using advanced bacterial engineering techniques, I plan to identify the commensal bacterial species that produce the metabolites that affect the action of the gut-brain axis through the modulation of the intestinal metabolite-sensing cells. Building on this, I will investigate how the intestinal commensal microbial species collaborates with intestinal metabolites sensors to respond to the internal status changes, including essential nutrient or chemical deprivation or overconsumption. Eventually, I will explore how the interactions between commensal gut bacteria and intestinal metabolite sensors affect the gut-brain axis and direct the behavioral shift to increase survival amid a challenging nutritional and metabolic crisis in flies.
Aim 3. To identify the metabolite-sensing system that detects the dietary microbial factors
Figure 3. A schematic to identify an external and internal gut microbial factors sensing systems in flies.
Having a commensal gut microbiota benefits animal survival by boosting immunity, improving digestion, and supplying essential metabolites. However, the commensal microbes offer not only these benefits but also direct behavioral changes in animals. A previous study reported that a specific metabolite exclusively made by the gut microbial species evokes locomotion changes by affecting the neuronal system in the fly brain (Schretter et al., 2018, Nature). Although several studies have shown that the commensal microbes provide significant help in survival, it is still largely unknown how the animals control their feeding behavior to have a better gut bacterial flora to increase survival. I have investigated how animals shift their feeding behavior to adapt to external and internal environmental changes and found that they actively sculpt their behavior according to those conditions to maximize survival. In my previous study, I showed the mechanism by which the nutrient-sensing neurons in the brain detect the circulating glucose level and control their behavior to maintain energy homeostasis. However, it is largely unknown how the animals detect the molecules that help establish a better intestinal microbial flora and switch their food choice, despite the importance of the balanced gut microbiota for a healthy life. Based on this, I hypothesize that the external odorant or taste receptors or internal metabolite-sensing cells in the brain or intestine detect the dietary microbial factors and switch the food choice to choose the food that helps establish better intestinal microflora (Figure 3). Moreover, those sensing systems can be specifically activated when animals have an unsuitable commensal microbial environment. The gut microbial factors indicate that the specific metabolites made by gut commensal bacteria, dietary fibers, and specific chemicals help construct the balanced gut microbial flora. I will test this hypothesis whether axenic flies prefer the food that contains the excreta from the healthy flies rather than the excreta from the axenic flies to enhance the quality of their gut microbial flora. After this confirmation, I plan to test whether the external odorant or taste sensing systems can detect the gut microbial factors in excreta. Recent studies imply that mosquitoes sense human skin cues and prefer a human with a more attractive skin odor derived from the human skin microbiota. Based on this study, I suppose that animals can sense the metabolites made by gut microbial species and change their behavior according to their internal status. Using the surgical removal of antenna or genetic knockout of odorant or taste receptors, I will test whether sensing the gut microbial factors using the external sensory receptors is essential for choosing the food containing the excreta of healthy flies in axenic flies. Additionally, I plan to find an internal sensing system in the brain or the gut to detect the gut microbial factors by expanding my research in Aim 2. Then, I will specify the chemicals only in the food chosen by axenic flies using ultrasensitive mass spectrometry. After identifying the candidate chemicals that drive the behavioral switch of the axenic flies, I will investigate the specific external or internal metabolite-sensing systems that detect those chemicals in the fly antenna, proboscis, midgut, or brain using an advanced genetic technique. Finally, I will characterize the physiological property of those gut microbial factor sensing cells. Then I will specify their downstream neuronal circuits that evoke the behavioral switch to establish a balanced gut microbial flora using updated genetics, physiology, and in vivo calcium imaging techniques.
Summary
To understand the metabolic control in animals, it is important to identify the communications between the brain and intestinal system and how those interactions are affected by the gut microbial factors. My research program will use advanced bacterial engineering, fly genetics, and calcium imaging approaches to investigate the functional connection between gut microbiota and the gut-brain signaling axis that controls feeding behavior and metabolism. Eventually, I will extend my studies to establish the metabolic functions of gut microbiota to maintain energy homeostasis and develop practical therapeutic approaches with the knowledge of the microbiome-gut-brain axis.


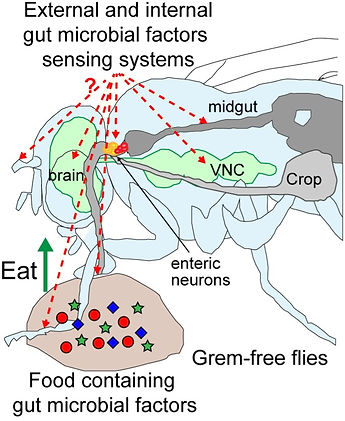
Research in Behavioral Neurophysiology Lab at Ewha
In an effort to gain a better understanding of Gene Transcription Enhancers, I have recently begun to use a new technique to investigate the organization and functionality of the diverse parts of my experimental model. I am currently looking to expand this work by collaborating with other labs who have the facilities and prior experience to investigate this project further.